The synthesis of thiophene-fused aromatic belts
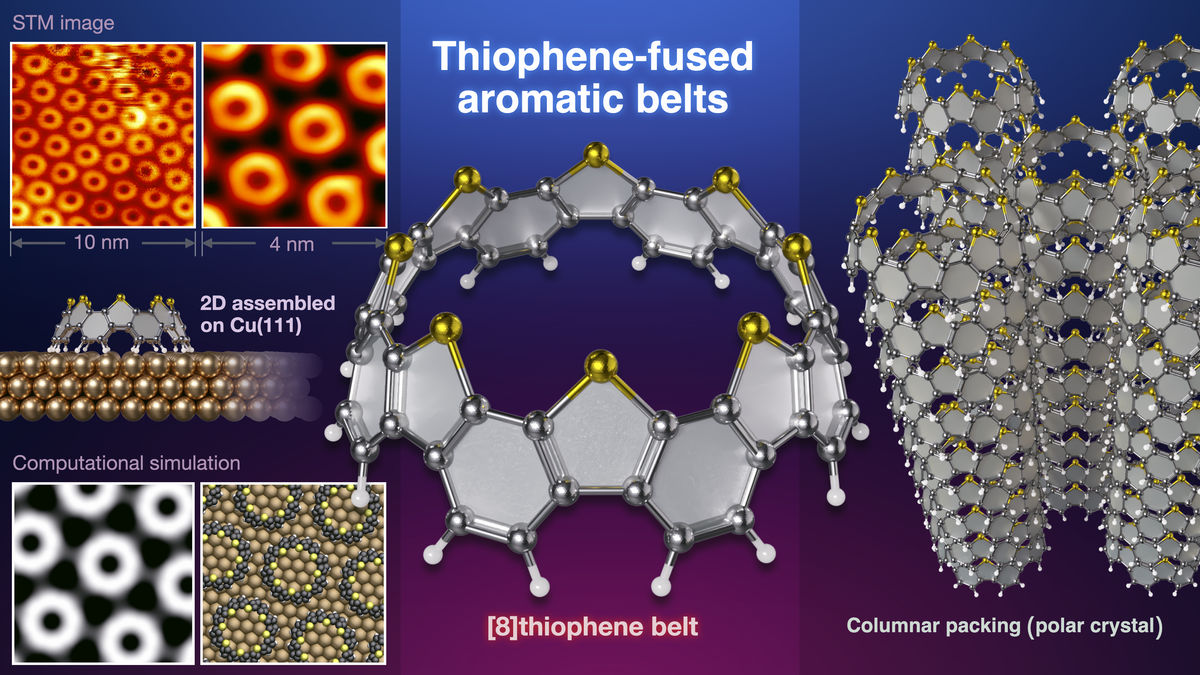
An international research group consisting of Chief Scientist Dr. Kenichiro Itami (RIKEN, Japan), Associate Professor Akiko Yagi (Nagoya University, WPI-ITbM, Japan), Prof. Harry Mönig (University of Münster, Germany), Prof. Saeed Amirjalayer (University of Heidelberg, Germany), and Prof. Nobuo Kimizuka (Kyushu University, Japan) has announced the first synthesis of thiophene-fused aromatic belts (thiophene belts), which are aromatic belts[1] incorporating thiophene[2] within its framework. The structural features of thiophene belts, including unidirectional columnar stacking with dipole moment in crystals, two-dimensional layer assembly on metal surfaces, and long-lifetime phosphorescence, are uncovered.
These unique properties of the thiophene belt are expected to lead to a variety of applications, including optoelectronic devices and polar materials.
The research was published in the online edition of the British scientific journal Nature Communications, dated 3rd February.
Introduction
Aromatic nanobelts, whose structures can be regarded as short carbon nanotubes[3], are a group of compounds that have attracted the interest of theoretical chemists as well as synthetic and physical chemists for nearly 70 years. Starting with the synthesis of a carbon nanobelt(ref1) reported by Dr. Itami in 2017, many research groups have now reported various carbon nanobelts and related aromatic nanobelts. These aromatic nanobelts have potential applications in fields such as organic electronics and supramolecular chemistry, and it has recently become clear that nanobelts can function as single-molecule devices(ref2). In the field of organic electronics, fused ring thiophenes are widely used as the basic backbone of p-type organic semiconductors[4], molecular conductors and light-emitting materials. Therefore, thiophene-fused nanobelts, in which aromatic nanobelts and fused ring thiophenes are fused, are molecules expected to become next-generation materials.
Dr. Itami and his colleagues thought to incorporate the thiophene moiety by applying a one-step sulfur cross-linking reaction to the carbon-fluorine bonds of partially fluorinated cycloparaphenylene(ref3), a carbon nanoring[5] they had developed independently.
(ref1) "Synthesis of a carbon nanobelt" Guillaume Povie, Yasutomo Segawa, Taishi Nishihara, Yuhei Miyauchi, Kenichiro Itami, Science 2017, 356, 172-175.
(ref2) "Highly efficient charge transport across carbon nanobelts" Junfeng Lin, Shengda Wang, Fan Zhang, Bowen Yang, Pingwu Du, Chuanfeng Chen,Yaping Zang, Daoben Zhu, Sci. Adv. 2022, 8, eade4692.
(ref3) "Half-substituted fluorocycloparaphenylenes with high symmetry: synthesis, properties and derivatization to densely substituted carbon nanorings" Hiroki Shudo, Motonobu Kuwayama, Yasutomo Segawa, Akiko Yagi, Kenichiro Itami, Chem. Commun. 2023, 59, 13494-13497.
Methods and results
Before the synthesis of the thiophene belts, the structure and strain energy of the designed thiophene belts were simulated by computational analysis using Okazaki Research Facilities, Research Center for Computational Science (Fig. 1A). The structure of the thiophene belt consists of a repeating structure of benzene rings[6] and fused thiophene rings, and thiophene belts were found that the shape changes with the number of units (n) of this structure. The shape is expected to change as n increases, in this order: cone-shaped (cone sliced in a circle), flat, and saddle-shaped. The strain energy was found to gradually decrease with the change from cone to flat shape, and to increase slightly in the saddle shape. In the present study, an attempt was made to synthesize thiophene belts with cone-shaped geometries.
Dr. Itami and his colleagues synthesized their own partially fluorinated cycloparaphenylenes (F16[8]CPP, F18[9]CPP) as precursors. Thiophene belts consisting of eight and nine constituent unit units were synthesized efficiently and in a short step by applying a sulfur cross-linking reaction to these carbon-fluorine bonds (Fig. 1B).
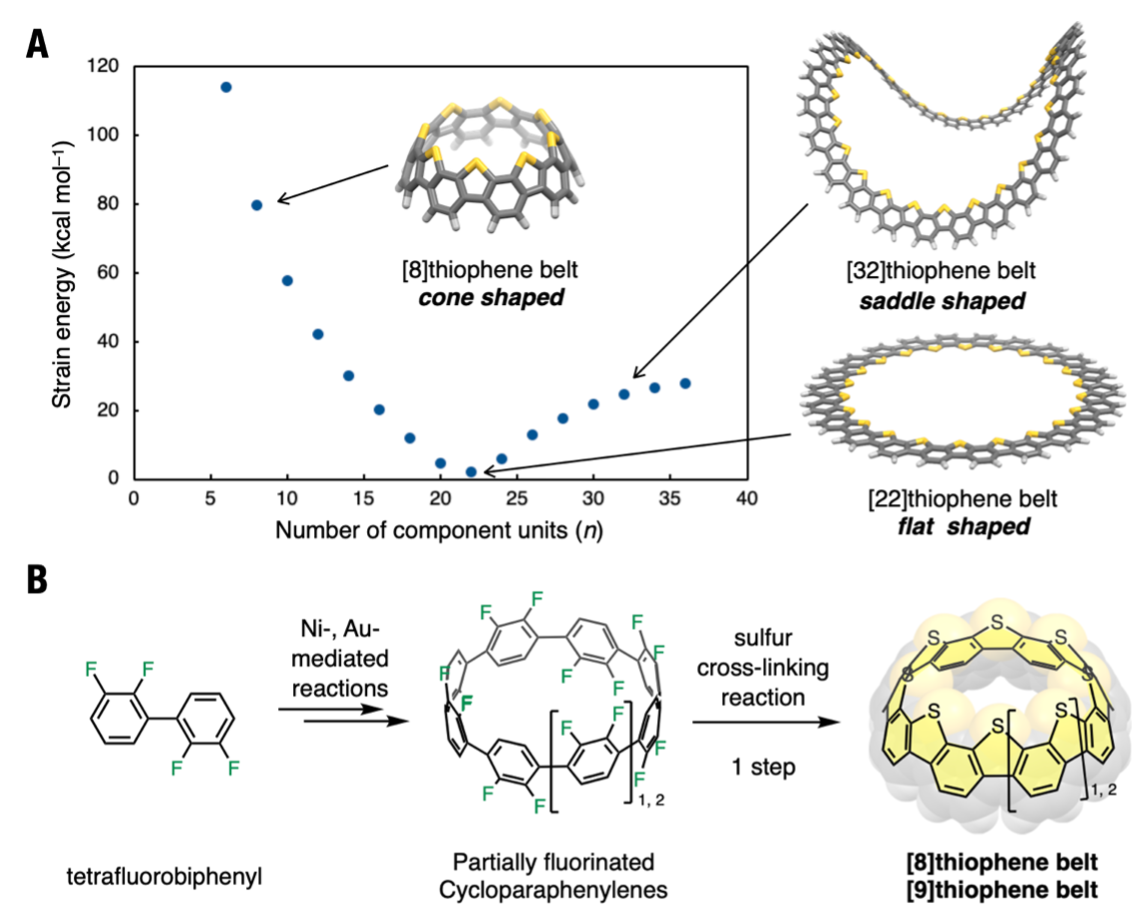
(A)Strain energies and structures of thiophene belts were simulated by calculation. The shape of thiophene belts depends on sizes of belt; n (number of benzene rings), we synthesized con-shaped structures in this work
(B)Synthetic scheme of thiophene belts. Tetrafluorobiphenyl was converted into nanobelts via partially fluorinated cycloparaphenylenes.
X-ray crystallography[7] determined the structure of the thiophene belts and revealed that they have large dipole moments[8] derived from their structures (Fig. 2A, B). As shown in Fig. 2C, the thiophene belts also have the interesting property of building columnar structures in the crystal with all the molecules aligned in the same orientation. When crystallization was investigated in several solvents, similar molecular arrangements were observed in all the solvents in which crystallization was carried out, suggesting that the thiophene belts, which have dipoles in a single molecule, are polar crystals with an aligned molecular arrangement.
In addition, investigation of the photophysical properties of the thiophene belt revealed absorption, fluorescence and phosphorescence at low temperatures of -196 °C, reflecting the rigidity of the thiophene belt. Time-resolved phosphorescence measurements by Prof. Nobuo Kimizuka and colleagues in Kyushu University, have revealed that the phosphorescence is long-lived at low temperatures.
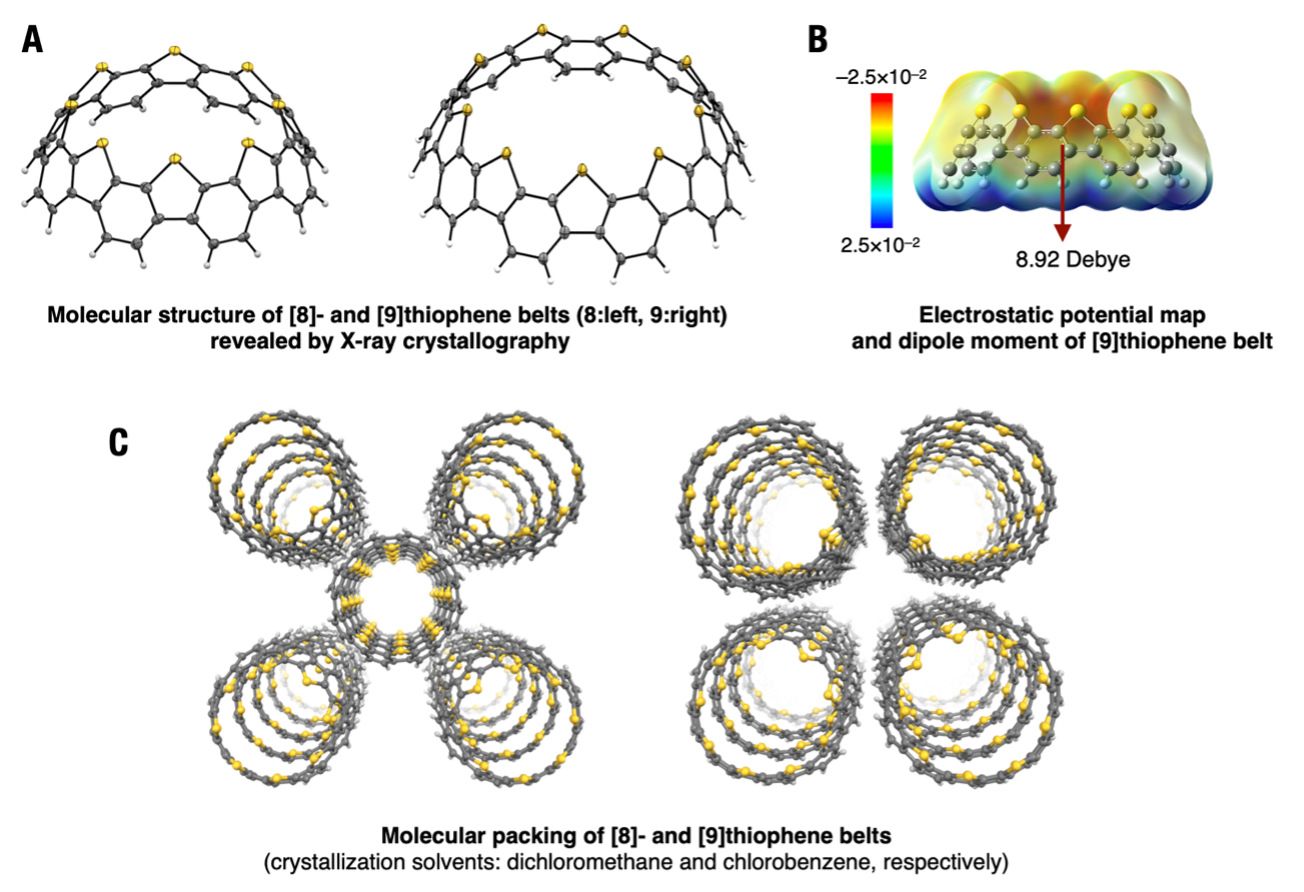
(A)The structure of thiophene belts which was revealed by X-ray crystallography. Carbon, sulfur, and hydrogen atoms were drawn in gray, yellow, and white colors, respectively.
(B)Electrostatic surface potential maps and the value of dipole moment. The dipole moment is due to the sulfur atoms, which cluster only on the upper side of the molecule, a large value for a single molecule.
(C)Molecular packing of thiophene belts in a crystal. All thiophene belts are aligned in the same direction and arranged in columns, and the crystals themselves are polar.
As the thiophene belts are a unique structure with sulfur atoms gathered only on the upper side and the interaction with the metal surface was of interest, the arrangement behavior on the metal surface was investigated using scanning tunneling microscopy[9] by Prof. Harry Mönig and colleagues in University of Münster. The metals used were gold (Au(111) surface) and copper (Cu(111) surface). Thiophene belts were observed aligned in one dimension against the steps created on the gold surface, while on the copper surface they were observed as single molecule-thick sheets assembled in two dimensions. Using simulations of microscopic images by Prof. Saeed Amirjalayer and colleagues in Heidelberg University, the state of the thiophene belt on metal surfaces has been clarified. On the gold surface, the thiophene belts were stable when assembled in the step area with the sulfur atoms facing downwards, while on the copper surface they were stable when assembled with the sulfur atoms facing upwards.
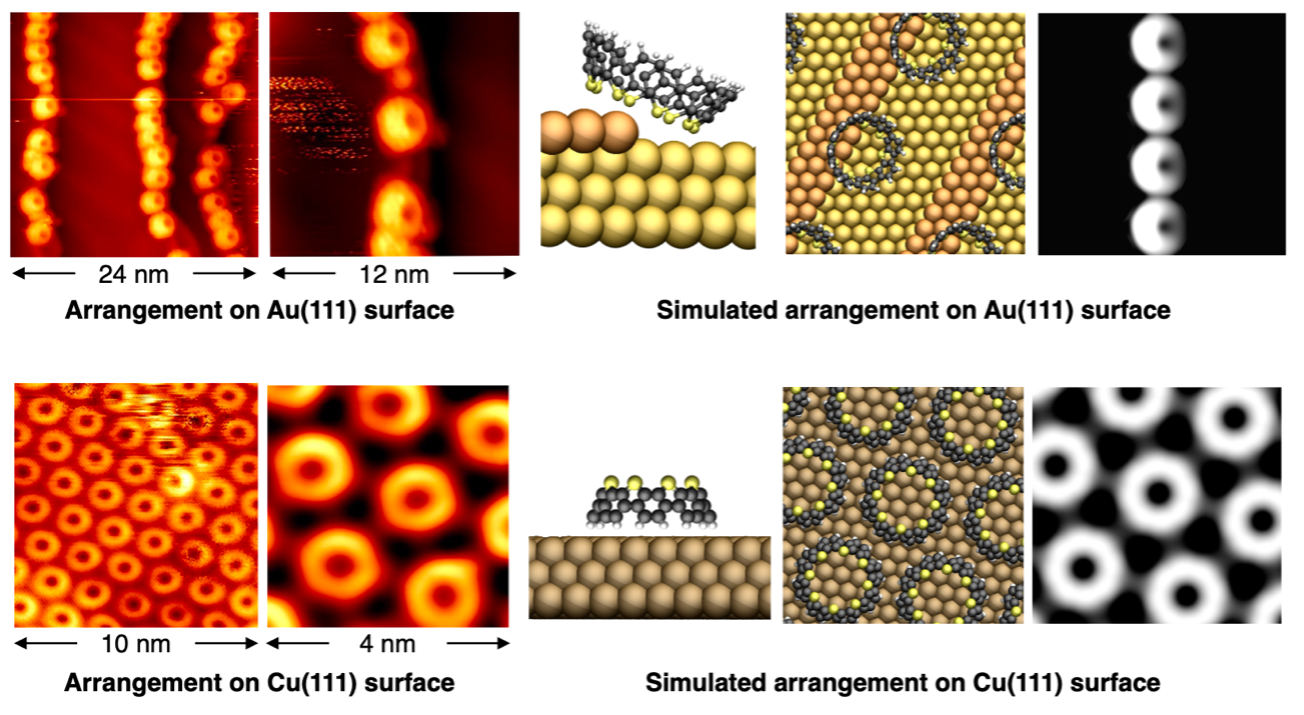
Fig 3. Arrangement of thiophene belts on a metal surface by scanning tunneling microscopy and an image of the arrangement simulated by computational science. On the gold surface, the molecules are aligned in one dimension and sulfur downwards, while on the copper surface, the molecules are aligned in two dimensions and sulfur upwards.
Future Expectations
In this work, thiophene belts with a unique structure incorporating a thiophene moieties were synthesized efficiently and in a short step. X-ray crystallography not only revealed the structure, but also the unique columnar molecular arrangement pattern in the crystal and its polar crystalline nature, while spectroscopic measurements revealed the long-lived phosphorescent luminescence was revealed. International collaborations have also revealed the arrangement patterns on gold and copper metal surfaces in the thiophene belt.
The thiophene belts synthesised in this study not only have potential applications as polar materials, but also as optoelectronic devices in the future, as they contain fused ring thiophenes, which are widely used in the field of organic electronics.
Appendix
[1] aromatic belt
A molecule consisting of aromatic rings connected in a tubular shape and having a rigid structure; the carbon nanobelts synthesised by Itami in 2017 are also included in the aromatic nanobelts.
[2] thiophene
Organic molecules with a pentagonal structure (called a five-membered ring) containing one sulfur atom. Aromatic compounds with a six π-electron system having a similar number of π-electrons as benzene in appendix[5].
[3] carbon nanotube
A type of carbon substance called nanocarbon, a tubular compound with a nanometer-sized diameter, first discovered by Dr. Sumio Iijima in 1991.
[4] p-type organic semiconductor
Among semiconductors, those that transport holes are known as p-type. Semiconductors based on organic molecules are also attracting attention as mechanically flexible and lightweight materials.
[5] carbon nanoring
A group of molecules consisting of aromatic rings connected by a single bond to form a ring.
[6] benzene rings
An organic molecule composed of six carbon atoms. The regular hexagonal carbon skeleton is called a benzene ring. The planar structure is the most stable; when curved, it has strain energy.
[7] X-ray crystallography
A technique to reveal the molecular structure and its arrangement in a single crystal by irradiating X-rays on the crystal and analyzing the diffraction pattern.
[8] dipole moment
When electronegativity differs between sulfur and carbon atoms, as in the case of sulfur and carbon atoms, intramolecular polarization occurs, which is called a dipole. The degree of polarization is expressed by the dipole moment.
[9] scanning tunneling microscopy
A type of microscope that detects weak tunneling currents by bringing a probe close to the sample. It has enough resolution to measure a single molecule as in this case.
Information
Title | Thiophene-fused aromatic belts |
---|---|
Author | Hiroki Shudo, Philipp Wiesener, Elena Kolodzeiski, Kiichi Mizukami, Daiki Imoto, Harry Mönig, Saeed Amirjalayer, Hirotoshi Sakamoto, Henning Klaasen, Bart Jan Ravoo, Nobuo Kimizuka, Akiko Yagi, Kenichiro Itami |
Journal | Nature Communications |
DOI | 10.1038/s41467-025-55896-w |
Date | 2025.2 |